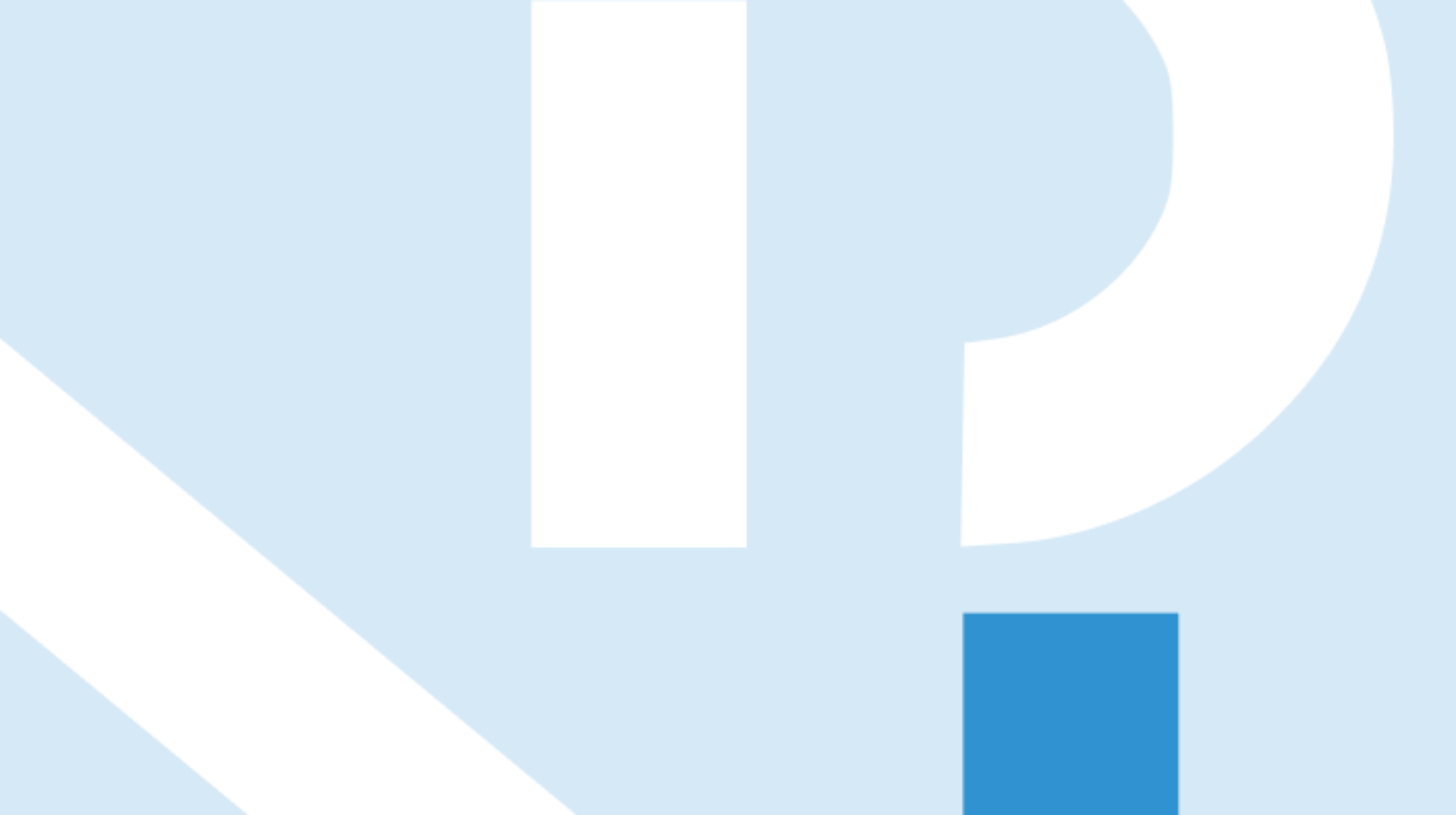
The role of mechanical force in physiology is becoming increasingly recognized
as an important factor in the human health. Mechanical force affects the
function, the organization and the remodeling of organs and tissues in the
human body, which are processes triggered by events occurring at the single-
molecule level. In the same way mechanical forces are actively present during
disease, and their involvement is calling the attention of the scientific and
medical communities. Among diseases, the pathogenic infections are not exempt
of mechanical forces, especially during the pathogen attachment to human
tissues where surface proteins from both the pathogen and the host interact
with each other. Currently, the traditional treatments against infections are
facing the challenge of the bacterial antibiotic resistance and the lack of a
cure for some pathogenic diseases of worldwide spread. In this sense, the
pharmacological targeting of the mechanical attachment of the pathogen at the
single-molecule level is seen to be a new approach to fight infections. This
new point of view enables the design and discovery of new drugs, and the new
fields of Mechanomedicine and Mechanopharmacology provide a suitable framework
for the development of new treatments against pathogenic attachment. Although
the last years have witnessed an increasing knowledge regarding single-
molecule mechanics, little is known about the mechanical features of the
proteins used by pathogens for attachment. Hence, basic research is needed to
unravel the mechanics of these molecules and to provide a basis from where a
pharmacological treatment can be developed.
With this goal, my thesis has focused on the study of the nanomechanics of
cell-surface proteins involved in viral and bacterial adhesion. Specifically I
have studied the proteins used by uropathogenic Escherichia coli to attach to
the urinary tract, and the human protein receptor CD4 used by Human
Immunodeficiency Virus (HIV) for recognizing and infecting immune cells.
We hypothesized that bacterial proteins display a high mechanical resistance
based on their adhesion function in the urinary tract. For the CD4 protein it
was hypothesized that its mechanical extension is related with the Human
Immunodeficiency Virus infection since this process requires the spatial
rearrangement of many elements for bringing the virus closer to the cell
membrane. Many disciplines and techniques are involved in this thesis but the
most important technique applied for the direct manipulation of single protein
molecules is single-molecule force spectroscopy.
In the first part of this thesis the mechanical resistance of the proteins
that build the attachment organelle of uropathogenic Escherichia coli was
studied. This extracellular structure is called type I pilus, a filamentous
appendage composed of hundreds of proteins used during urinary tract
infection. We found that pilus proteins show not only a remarkable resistance
to mechanical unfolding but also that they follow an unfolding hierarchy
pattern tightly connected to their position in the pilus structure. Our
results reveal that conserved disulfide bonds in each of the pilus proteins
are a crucial feature for their mechanical stability. Besides unraveling its
nanomechanical architecture, the biogenesis of the pilus was studied wherein
we recreated in vitro the same events that take place during the maturation
and folding of the proteins previous to their incorporation into the pilus.
During our investigation we found that the enzyme DsbA involved in disulfide
bond creation during the first step of pilus proteins maturation shows an
additional pronounced chaperone effect, which helps to fold to pilus proteins
in a greater extent than the putative real chaperone of this system. With
these findings we provide for the first time a detailed description at the
single-molecule level of the type I pilus mechanical architecture and
biogenesis.
In the second part of this work the nanomechanics of the lymphocyte T cell-
surface protein CD4 were studied. The CD4 protein is used by the HIV particle
to attach to these cells. The subsequent events for viral infection require
the approaching of the particle to the cell membrane to interact with other
proteins meanwhile attached to CD4. We have discovered that the first two
domains of CD4, D1D2, are able to unfold at low forces. We suggest that this
is the range of forces that a viral particle could produce due to thermal
motion. In this scenario CD4 would act as a shock absorber in which its
domains can unfold at low forces by the virus, therefore increasing the length
of the tether and allowing the virus to explore the cell membrane looking for
the other proteins required for viral fusion. Also the regulation by
oxidoreductase enzymes of the disulfide bonds of CD4 during HIV infection is
important for the infectivity of the virus. We demonstrated that only the
mechanical unfolding of CD4D1D2 makes possible the access of the
oxidoreductase enzyme to the buried disulfide bonds, supporting the idea that
the viral particle could unfold CD4 in vivo. A mathematical model was
developed based on the infectivity of cells expressing engineered variants of
CD4 showing different tether lengths. The infectivity increases with the
length of the tether, and the model allows interpreting that the in vivo
infection would require the extension of CD4. Finally the effect of
Ibalizumab, an antibody known to be a good neutralizer of HIV infection was
tested. This antibody’s epitope is placed between CD4D1 and CD4D2 domains
and the exact mechanism for its potent neutralizing effect is unknown. We have
found that the mechanical stability of CD4D1D2 is increased in the presence of
Ibalizumab, suggesting that the binding of the antibody hinders the extension
of CD4 by the virus and this avoids the subsequent events of the infection.
This supports again that the mechanical extension of CD4 is mandatory for
successful virus infection. Altogether our results suggest that CD4
mechanochemical extension may play a critical role during HIV infection.
In the light of these findings, we think that our results could help to
develop new therapeutic strategies oriented to alter the nanomechanics of
proteins involved in pathogenic attachment, in the context of the new fields
of Mechanopharmacology and Mechanomedicine. In the case of bacteria, the use
of molecules which disrupt the strength of the pilus proteins would circumvent
the current limitations of traditional antibiotic treatments. For HIV, we
suggest that an increased mechanical stabilization of CD4 receptor induced by
antibody binding could prevent the infection or diminish its chances of
success.
**Supervisor** : R. Perez-Jimenez