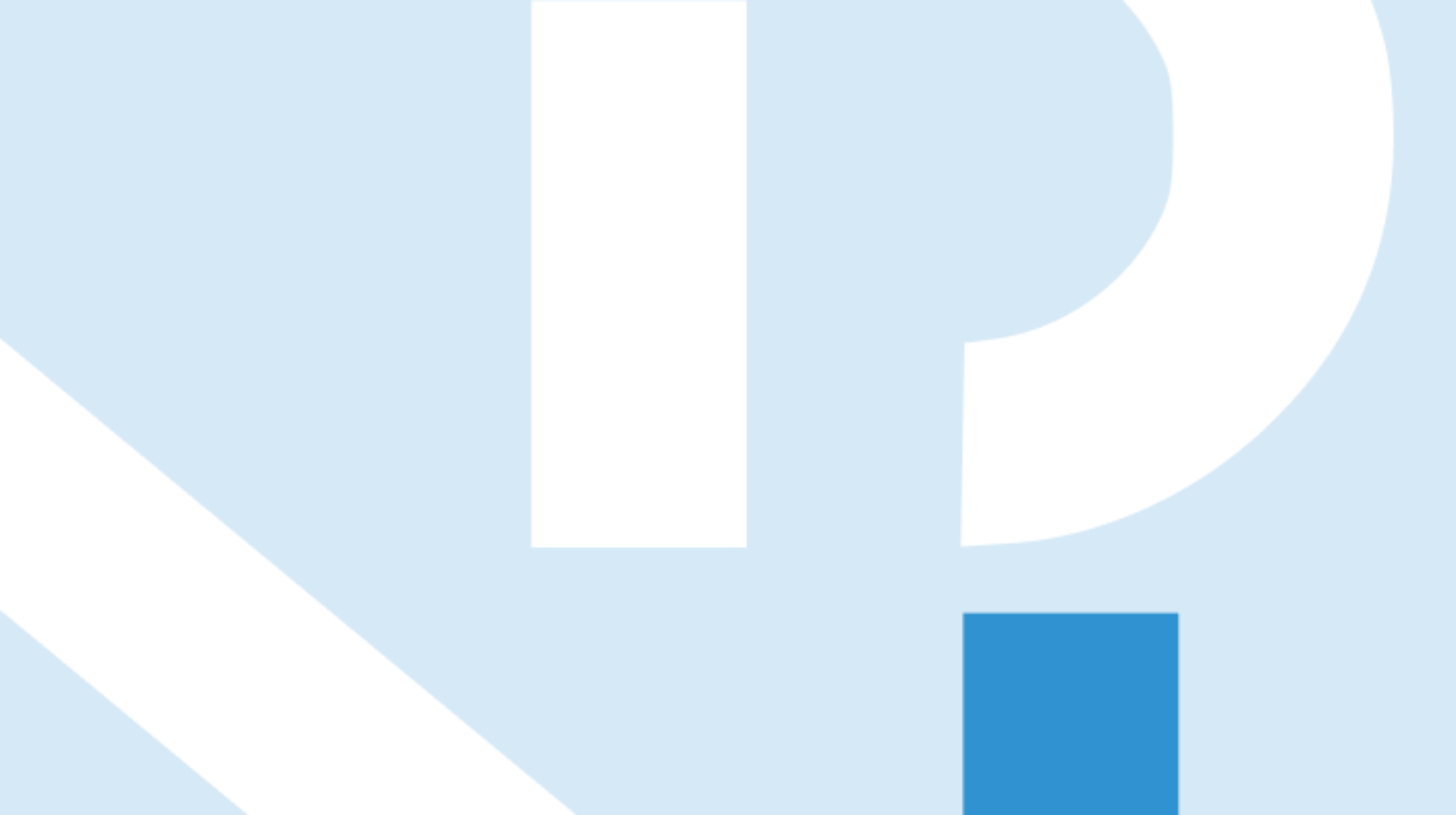
This thesis collects the most relevant part of the work carried out during my
Ph.D. project, started on the 6th of February 2014 in the Nanomagnetism group
under the supervision of Prof. Paolo Vavassori. The original results contained
in the thesis are all connected to the properties of interacting ferromagnetic
nanostructures. A peculiar effect related to interactions is frustration,
namely the inability of a physical system to simultaneously satisfy (i.e.
energetically minimize) all the pairwise interactions within it. This
definition means there can be various (non-trivial) configurations of the
considered system which are associated to the same energy. When this happens,
the system ground state results to be degenerate, leading to the appearance of
interesting features, e.g. a finite value for the zero-point entropy. A
classical example is given by the proton arrangement in water ice. Moreover,
the same behaviour has been found in other condensed matter compounds, in
particular regarding the atomic spin arrangement. These materials constitute
the class of spin ice systems. However, in 2006 [1], a new class of
metamaterials has been introduced: the artificial spin ice (ASI) systems. They
are two-dimensional realization of bulk spin ice systems that can be
fabricated by electron beam lithography, since they are nothing else than
arrays of interacting ferromagnetic nanostructures. One of the most
interesting processes to be studied in ASI systems is the ground state
formation after heating up and cooling down the system to room temperature
[2]. Depending on the parameters characterizing both the whole ASI system and
its constituent nanostructures, the ground state can finally be reached or
not, since (i) the material, (ii) the nanostructure arrangement in the array,
(iii) the nanostructure shape and (iv) the temperature do have an impact on
the process. Therefore, we took into account all these factors by designing
and implementing a multiscale simulation scheme which is able to combine
micromagnetic simulations for the description of the thermal behaviour of
magnetic nanostructures (hundreds of nanometers, tens of microseconds) with
kinetic Monte Carlo calculations for considering the response of the whole
system (tens of micrometers, hundreds of seconds). The results derived form
our approach have been compared to data available in literature [3] to check
the validity of our arguments. Besides the above described multiscale
simulation scheme, we also exploited the base knowledge of our research group
(i.e. magnetism and plasmonics) for conceiving a non-standard heating
technique for ASI systems: the selective and localized thermoplasmonic heating
[4] of magnetic nanostructures. The transfer of energy from an electromagnetic
wave to a metallic nanostructure can be tremendously effective when the
localized surface plasmon resonance is excited, whereas this transfer can be
almost turned off when the nanostructure electron system is out of resonance.
Moreover, at a fixed incident wavelength, the energy transfer can be modulated
according to the direction of linear polarization with respect to the
nanostructure axes, since the resonance frequency depends on the axes aspect
ratio (heating selectivity). Eventually, a great advantage of working with
light beams is the fact that the sample area in which energy is delivered can
be controlled by simply focusing or defocusing the beam itself, down to a
diameter of 1 micrometer (localized heating).
[1] R. F. Wang et al., Nature 439, 303 (2006).
[2] J. M. Porro et al., New J. Phys. 15, 055012 (2013).
[3] A. Farhan et al., Phys. Rev. Lett. 111, 057204 (2013).
[4] G. Baffou et al., ACS Nano 7, 6478 (2013).