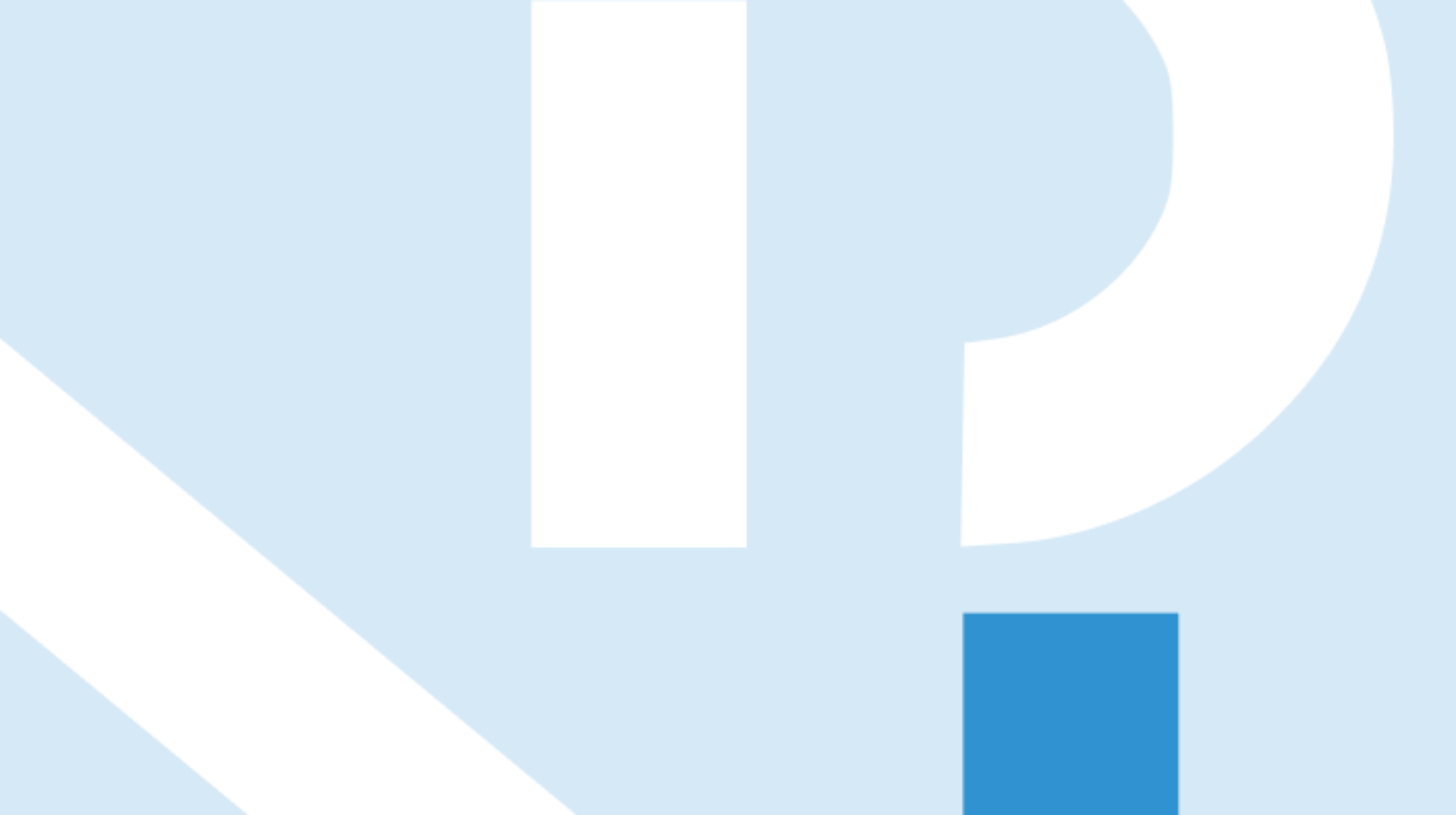
**Could silicon enable a quantum computing revolution?**
M. Fernando Gonzalez-Zalba
Lead Quantum Engineer and UKRI Future Leader Fellow at Quantum Motion
Technologies fernando@quantummotion.tech
The silicon metal-oxide-semiconductor transistor is the workhorse of the
microelectronics industry. It is the building block of all major electronic
information processing components such as microprocessors, memory chips and
telecommunications microcircuits. By shrinking its size generation after
generation, the computational performance, memory capacity and information
processing speed has increased relentlessly. However, the process of
miniaturization is bound to reach its fundamental physical limits in the next
decades.
New computing paradigms are hence paramount to overcome the technical
limitations of silicon technology and to continue increasing the computation
performance beyond simple multi-core approaches. Quantum computing offers
exponential speed-up over several classical algorithms, and it is hence one of
the most sought-after alternatives to conventional computing. However, finding
the optimal physical system to process quantum information and scale it up to
the large number of qubits necessary to run useful quantum algorithms remains
a major challenge. Paradoxically, we are now starting to see that silicon
technology itself could offer an optimal platform on which to fabricate
scalable quantum circuits: Quantum computing with silicon transistors could
profit from the most established industrial technology to fabricate large
scale integrated circuits [1], a fact that facilitates the integration with
conventional electronics for fast data processing of the binary outputs of the
quantum processor [2,3]; all this offering long electron spin coherence times
[4], high-fidelity spin readout [5], and one- and two-qubit gates [6-8], the
basic physical requirements to build a quantum computer.
First, in this talk, I will review the field of silicon-based quantum
computing going from the basic physics that govern spin qubits in this
material, all the way to the technological implementation, the state-of-the-
art and the scaling challenges ahead. Secondly, I will present a series of
results on industry- manufactured silicon transistors at milikelvin
temperatures that show the technology could provide a platform on to which
implement electron spin qubits including our new spin measurement technique
that has enabled the first detection of electron spin dynamics in an industry-
fabricated device [5,9]. Then, I will present results on how digital, analog,
and quantum devices can be combined to perform readout at scale using time-
and frequency-multiplexing [3]. Finally, will show a roadmap of how the
architecture can be scaled up [10] and, if time allows, fundamental and
technological side effects of doing research in silicon quantum computing [11,
12].
**References **
[1] M.F. Gonzalez-Zalba, Nat Elect 4 872 (2021) [2] S. Schaal, Nat Elect 2 236
(2019)
[3] A. Ruffino, Nat Elect 5 53 (2022)
[4] M. Veldhorst, Nature, 526, 410 (2015)
[5] G.A.Oakes, arxiv2203.06608 (2022)
[6] J. Yoneda, Nat. Nanotech. 13 102 (2018)
[7] X. Xue, Nature 601 343 (2022)
[8] A. Noiri, Nature 601 338 (2022)
[9] V. N. Ciriano-Tejel, PhysRevX Q 2 010353 (2021)
[10] O. Crawford, arxiv2201.02877 (2022)
[11] L. Cochrane, Phys Rev Lett 128 197701 (2022)
[12] T. Lundberg, Phys Rev X 10 041010 (2020)
**Host:** J. M. Pitarke